Raman spectroscopy is a very effective technique for on-line measurements under various process and experimental conditions. However, many phase changes and other interesting reactions occur at high temperature and/or pressure.
Raman spectroscopy has been used for many years to measure important information in these extreme conditions, and it can even be used simultaneously for accurate temperature determination (Raman Thermometry). For such experiments, it is usually a non-contact technique, moreover, measurements can be carried out remotely, which reduces the risk of thermal damage to the measuring devices. Another option is Raman high-temperature immersion probes.
However, a challenge in non-contact high-temperature Raman measurements is often spectral interference caused by thermal emission from the sample. This can easily cover the Raman spectral bands, which are many times lower in intensity than thermal radiation, and reduce the signal-to-noise ratio already at temperatures exceeding approx. 500 °C, while process applications of molecular spectroscopy are even around 1600 °C – e.g. here.
The most common methods for limiting thermal interference with Raman spectroscopy include time-gated Raman spectroscopy, UV Raman spectroscopy (usually lasers below 300 nm, more here) and spatial filtering techniques. The least instrumentally and financially demanding technique for similar types of experiments is precisely time-resolved Raman spectroscopy (Time-Gated Raman with a pulsed excitation laser).
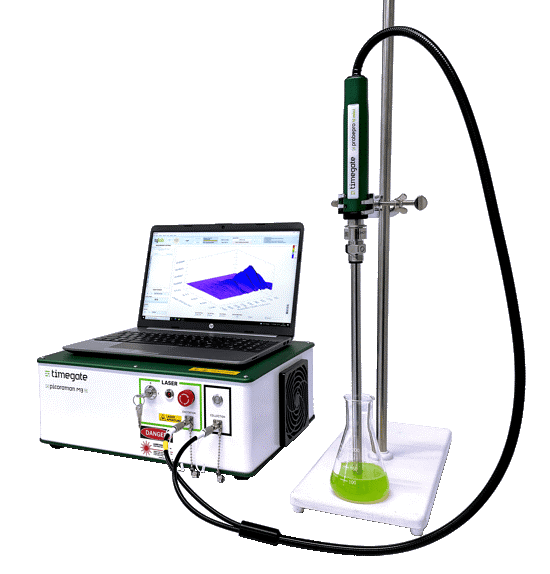
When measuring Raman spectra at high temperatures, the measured intensity on the detector is the sum of the Raman signal and thermal emission (fluorescence is not considered here). In order to achieve optimal results, we want to keep the ratio of the detected Raman scattering to the thermal emission (Thermal background – thermal BG) of the sample as high as possible. Picosecond time-resolved pulse techniques try to achieve this by using very fast, high-energy laser pulses and collecting data only during those pulses.
The figure below compares continuous wave (CW – traditional Raman) and pulse data collection in high temperature applications. This comparison also applies to other continuous interferences, e.g. sunlight.
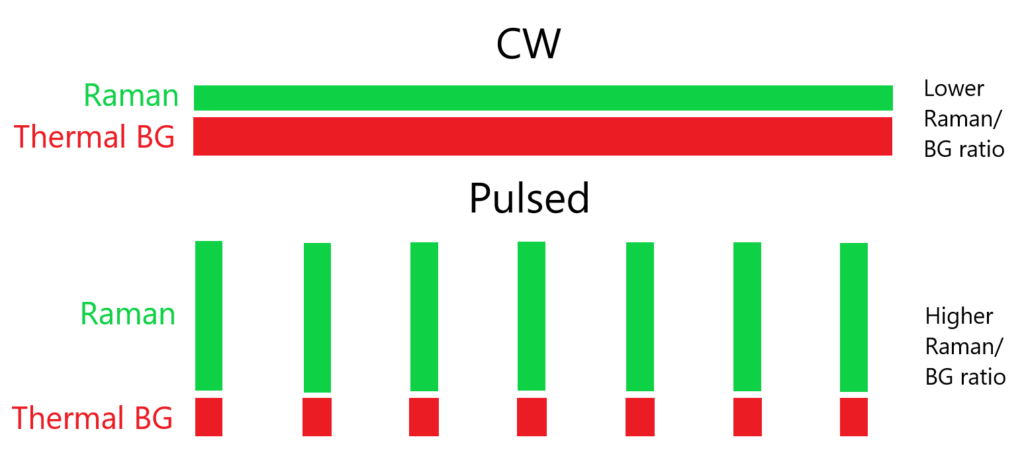
While classical Raman spectroscopy techniques collect information continuously, pulsed systems record data only during very short pulses. The resulting ratio of the Raman signal to the thermal background is then sufficiently high. Of course, high-power pulsed lasers can promote sample degradation, but this is not a problem for those that can withstand very high temperatures.
Another advantage of pulsed time-resolved techniques is that the thermal background can also be measured just before the pulse as a background. In this way, the measurement of this background can be repeated often and the result of this background measurement will not include any Raman responses, since the measurement takes place between excitation laser pulses. This can then simply be used to further read off the thermal effect. This technique was also used in the example in the following image:
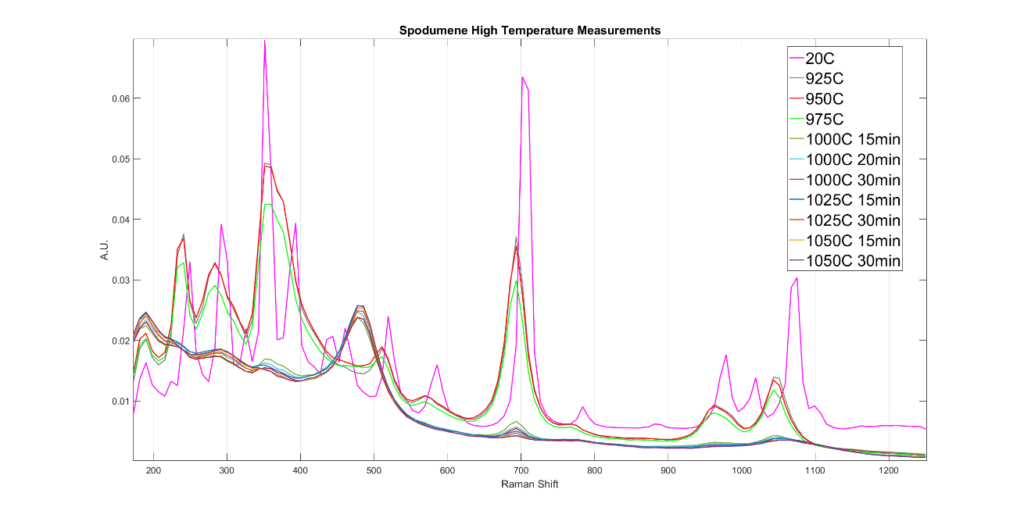